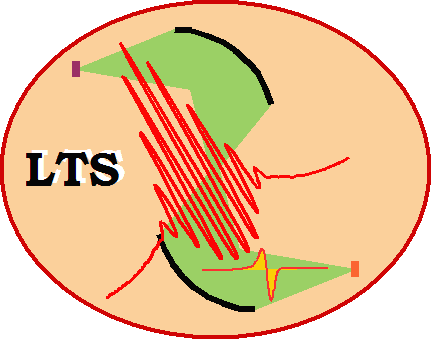
THz science and technology
Highlights
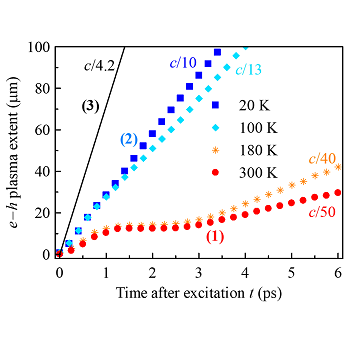
Ultrafast long-distance expansion of electron-hole plasma in direct bandgap semiconductors
Transport of charge carriers inside crystals is determined by their energy band structure which only permits velocities smaller than ~c/100 in known materials. We demonstrated that ultrafast and long-distance propagation of electron-hole plasma (velocities up to c/10, reaching more than 100 μm) is possible as a quite general result of fundamental electron-photon interaction in direct bandgap semiconductors upon strong pulse photoexcitation with low photon excess energy above the bandgap [PRL 130, 226301 (2023) & Nanophotonics 13, 1859 (2024)].
Electron-hole plasma expansion with velocities exceeding c/50 and lasting over 10 ps at 300 K was evidenced by time-resolved terahertz spectroscopy [1]. This regime, in which the carriers are driven over >30 μm is governed by stimulated emission due to low-energy electron-hole pair recombination and reabsorption of the emitted photons outside the plasma volume. At low temperatures a speed of c/10 was observed in the regime where the excitation pulse spectrally overlaps with emitted photons, leading to strong coherent light-matter interaction and optical soliton propagation effects.
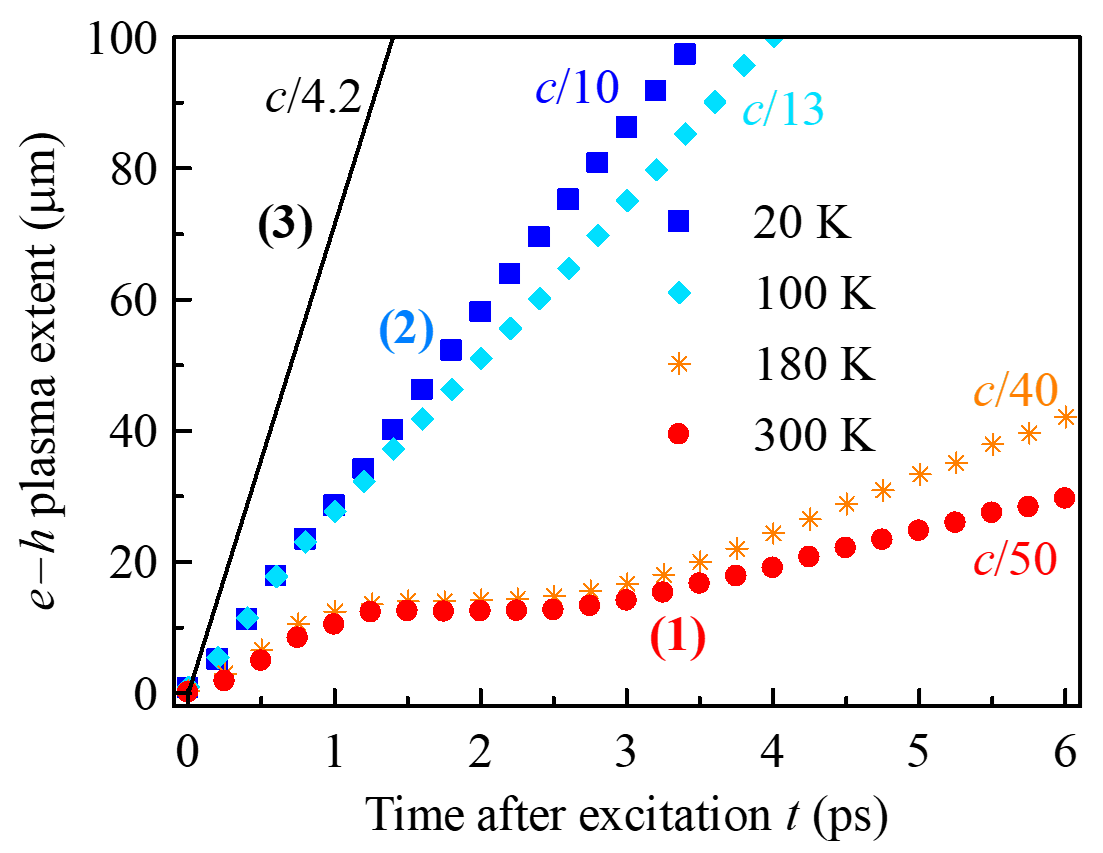
Figure:
Propagation of plasma as measured by THz pulse probing of photocarriers in GaAs shows that assistance of light can dramatically enhance
the charge propagation rates.
(1) In incoherent regime the plasma propagation is due to stimulated emission and reabsorption of photons;
(2) In coherent regime at low temperature the electron – photon interaction leads to Rabi dynamics and soliton formation and propagation;
(3) for comparison: group velocity of light in GaAs.
[1] T. Troha, F. Klimovič, T. Ostatnický, F. Kadlec, P. Kužel, and H. Němec, Ultrafast long-distance electron-hole plasma expansion in GaAs mediated by stimulated emission and reabsorption of photons, Phys. Rev. Lett. 107, 226301 (2023).
[2] T. Troha, F. Klimovič, T. Ostatnický, H. Němec and P Kužel, Photon-assisted ultrafast electron–hole plasma expansion in direct band semiconductors, Nanophotonics 13, 1859 (2024).
(show less)
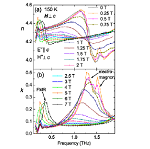
Electromagnon in the Y-type hexaferrite BaSrCoZnFe11AlO22
We investigated static and dynamic magnetoelectric properties of single crystalline BaSrCoZnFe11AlO22 which is a room-temperature multiferroic with Y-type hexaferrite crystal structure. THz and Raman spectra reveal an electrically active spin wave (electromagnon) below 300 K at ≈1.2 THz. We show that the electromagnon is activated due to the magnetostriction mechanism involving spin vibrations along the hexagonal axis.
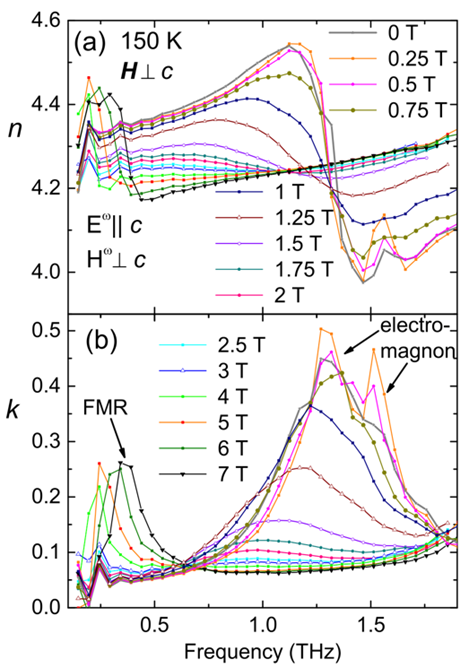
Figure: Electromagnon in THz spectra of BaSrCoZnFe11AlO22.
[1] J. Vít, F. Kadlec, C. Kadlec, F. Borodavka, Y.S. Chai, K. Zhai, Y. Sun and S. Kamba, Electromagnon in the Y-type hexaferrite BaSrCoZnFe11AlO22, Phys. Rev. B 97, 134406 (2018).
(show less)
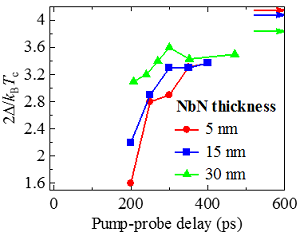
Departure from BCS response in photo-excited superconducting NbN films observed by terahertz spectroscopy
Ultrashort laser pulses can be used to induce transient exotic states of matter and cause phenomena of a vital interest such as room temperature superconductivity. We have focused on the photo-induced dynamics in niobium nitride (NbN) thin films [1] under strong excitation.
NbN is a prototypical BCS superconductor in the ground state. In the strong excitation regime the pump pulse immediately breaks all the Cooper pairs into separate quasiparticles. Subsequently, as the heat is dissipated out of the films towards the substrate, Cooper pairs start to recover. Using time-resolved terahertz spectroscopy, we characterized a series of NbN films with various thicknesses under such strong photoexcitation. Analysis of the photoconductivity spectra reveals that the recovery of Cooper pairs initially proceeds through the emergence of mutually isolated superconducting islands, which subsequently grow with increasing time towards a nearly percolated superconducting network. The superconductivity restoring is faster than the recovery of the superconducting gap to its equilibrium value. Most interestingly, experiments suggest that the profile of the density of states of strongly photoexcited films differs during the recovery process from the ones obtained for the thermal equilibrium at any temperature. This phenomenon is controlled by confinement effects within the films.
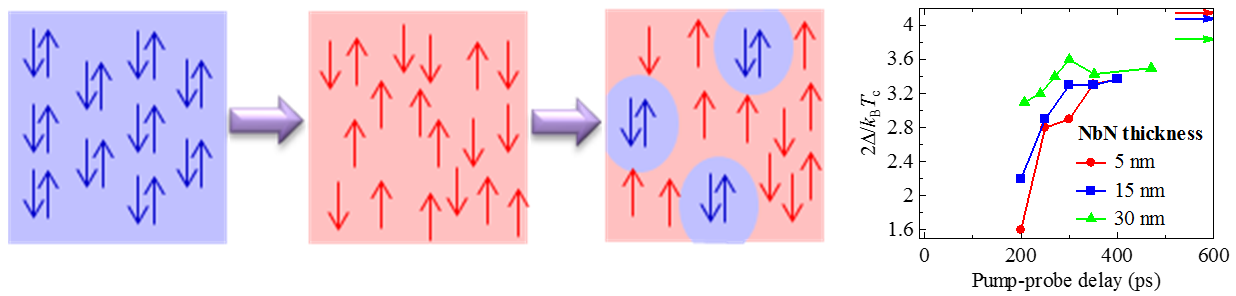
Fig. 1: Left panel: Photo-induced dynamics of quasiparticles (red) and Cooper pairs (blue). Right panel: Evolution of the superconducting gap 2Δ with time after photoexcitation. The arrows in indicate the gap widths in equilibrium.
[1] M. Šindler, C. Kadlec, P. Kužel, K. Ilin, M. Siegel, and H. Němec, Departure from BCS response in photoexcited superconducting NbN films observed by terahertz spectroscopy, Phys. Rev. B 97, 054507 (2018).
(show less)
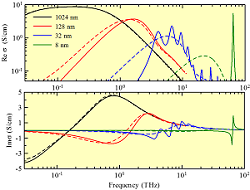
Quantum theory of terahertz conductivity of semiconductor nanostructures
In collaboration with T. Ostatnický of Charles University we participated to the development of the first theory of the quantum conductivity describing the transport in the terahertz spectral range, which does not contain internal contradictions. We have shown that the broken translation symmetry of the nanostructures induces a broadband drift-diffusion current which must be explicitly taken into account.
Usually, all the relevant charge carrier scattering processes
in the studied system are not known since their independent experimental determination is difficult.
The usual approach based on the Kubo formula introduces a phenomenological charge scattering rate
(or relaxation time) accounting for all the scattering processes.
This approximation is highly pertinent in the optical range.
However, the approach fails at low frequencies in nanocrystals in the regime
where the scattering rate is comparable to the probing frequency;
e.g., it always yields nonzero conductivity at zero frequency (dc regime)
even if the nanocrystals are mutually perfectly isolated.
We have shown
[1]
that the broken translation symmetry of the nanostructures induces
a broadband drift-diffusion current, which is not taken into account in the analysis
based on Kubo formula in the relaxation time approximation.
The proper introduction of this current removes all the contradictions,
fulfills the classical limit in the case of large nanocrystals and it is
at the origin of significant reshaping of the conductivity spectra up to terahertz or
multiterahertz spectral ranges. It is used for the interpretation of
temperature dependent photoconductivity spectra in various nanocrystal systems.
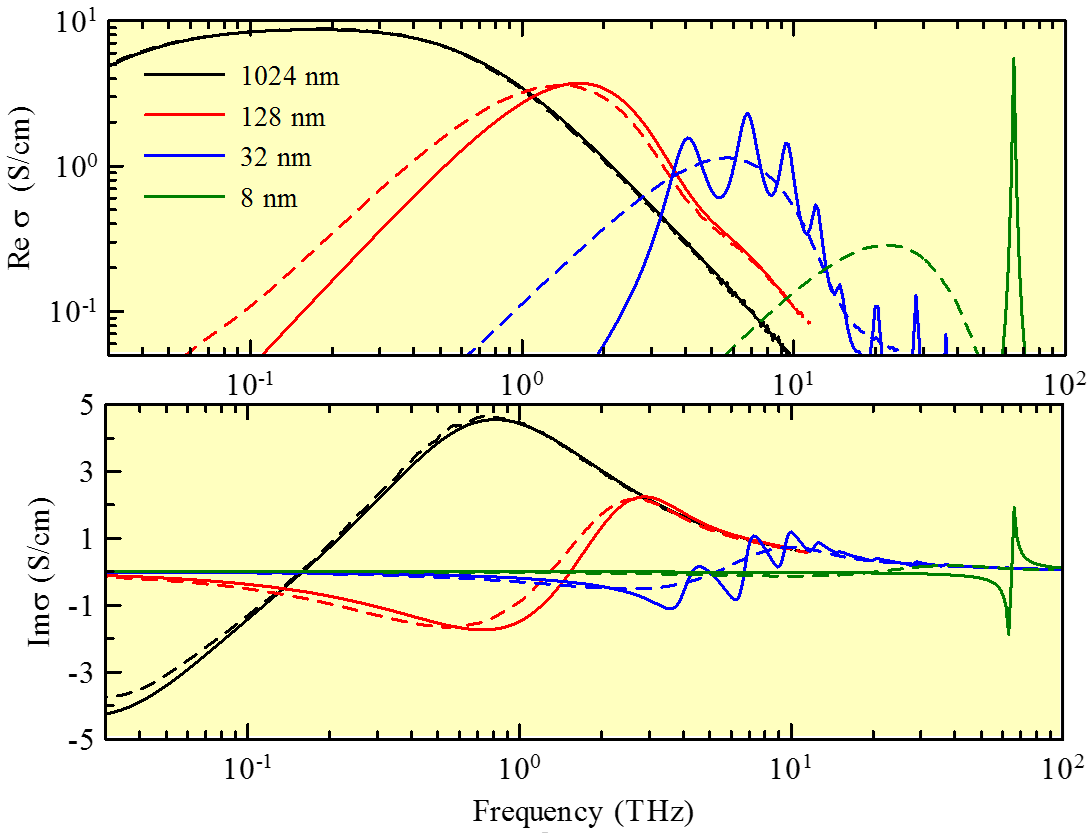
Fig. 1: Comparison of quantum (solid line) and classical (dashed line) conductivity in GaAs cube-shaped nanocrystals of selected sizes at 300 K and free carrier concentration of 1016 cm-3. Note the excellent agreement of quantum calculations with the classical ones for large nanocrystals (1024 nm).
[1] T. Ostatnický, V. Pushkarev, H. Němec, and P. Kužel, Quantum theory of terahertz conductivity of semiconductor nanostructures, Phys. Rev. B 97, 085426 (2018).
(show less)
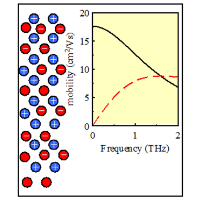
Picosecond charge transport in rutile at high carrier densities studied by transient terahertz spectroscopy
In rutile the charge carriers form polarons with high effective mass owing to the strong electron-phonon coupling. In this paper we studied ultrafast terahertz photoconductivity in rutile under strong optical excitation.
In this regime the polaron mobility depends on the polaron density;
as a consequence, highly complex transport phenomena occur on a picosecond scale
involving fine interplay between diffusion and recombination.
We developed a general model to disentangle these phenomena and
we determined dynamical properties of electron and hole polarons.
At room temperature internal degrees of freedom of polarons
are also observed through a dramatic increase of the transient permittivity
in photoexcited state
[
V. Zajac, H. Němec, and P. Kužel, Phys. Rev. B 94, 115206 (2016)].
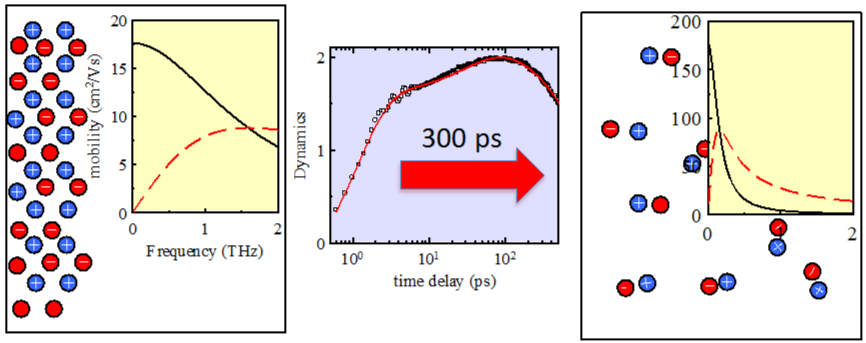
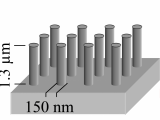
Ultrafast photoconductivity in semiconductor nanostructures
Time-resolved terahertz spectroscopy provides a useful insight into charge carrier motion in nanostructured semiconductors: it provides a contact-free access to local response of charge carriers inside nanostructures averaged over macroscopic volume of a sample. This is a very pertinent quantity for many applications of disordered materials which still have a larger potential than perfectly aligned nanostructures.
Straightforward fitting of the raw terahertz conductivity spectra by the Drude-Smith model, which was abundantly used in the literature, did not lead to a significant advance in an in-depth understanding of these phenomena. This is mainly because of the depolarization fields which build up in any inhomogeneous system. On the one hand, these fields reflect the sample morphology and their understanding in each particular system may provide new information about the nanostructure connectivity. On the other hand, the effect of unknown depolarization fields can hide or distort fingerprints of the nanoscopic transport.
Recently, we devoted a systematic effort to describe the depolarization fields in photoconductive nanostructures and to disentangle their effect from that of the local carrier response function [1].
- We proposed a general relation between the effective photoconductivity (measured in the THz experiment) and the microscopic response function. It is based on the general Bergman representation of effective medium theory for a two-component system with a single dominant depolarization factor. We have shown that our model describes both percolated and non-percolated samples and structures with complex percolation pathways [2].
- We solved the wave equation in an inhomogeneous sample and demonstrated that the transient sheet photoconductivity is directly measured in THz experiments for any spatial profile of the photoconductivity and any thin film sample morphology [1,3].
- The microscopic response function can be determined by Monte-Carlo calculations of the motion of a single carrier inside nanoparticles [4].
Analysis of experimental data within this framework allows us to uncover the nature of charge carrier transport at nanoscale and to assess the sample morphology in quite arbitrary nanostructured systems.
Based on this approach we proposed microscopic models of the response of partly or completely localized charge carriers in a number of systems including various TiO2 nano- and microparticle networks [2,5], in Sb-doped SnO2 nanoparticles [6,7], and in various nanocrystalline silicon systems [3,8], For example, in [9] we investigated a regular array of vertically aligned InP nanowires (Fig. 1). In this structure, photonic waveguiding effects inside nanowires and interferences of the excitation optical beam should have been properly taken into account to describe the sample photoexcitation. We were able to determine high transversal electron mobility in nanowires which, in comparison with the longitudinal mobility, indicates the presence of stacking faults in the growth direction (Figure 1).
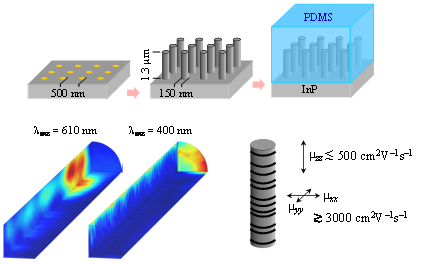
Figure: (a) Sketch of the preparation of the vertically aligned InP nanowires and scheme of the investigated structure. (b) Distribution of the excitation density in the nanowires for two different excitation wavelength λexc. (c) The transversal mobility (μxx, μyy) is considerably higher than the longitudinal one (μzz) which is limited by the stacking faults along the growth direction.
References
[1] P. Kuzel and H. Nemec, J. Phys. D: Appl. Phys. 47, 374005 (2014).
[2] H. Nemec et al., IEEE Trans. Terahertz Sci. Technol. 3, 302 (2013).
[3] V. Zajac et al., New J. Phys. 16, 093013 (2014).
[4] H. Nemec et al., Phys. Rev. B 79, 115309 (2009).
[5] S. A. Jensen et al., J. Phys. Chem. C 118, 1191 (2014).
[6] K. Peters et al., Chem. Mater. 27, 1090 (2015).
[7] K. V. Skoromets et al., J. Phys. Chem. C 119, 19485 (2015).
[8] H. H. Němec et al., Phys. Rev. B 91, 195443 (2015).
[9] C. S. Ponseca et al., Phys. Rev. B 90, 085405 (2014).
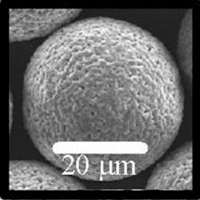
Nonmagnetic microspheres with resonant magnetic response
Metamaterials are artificial resonant composite structures formed by common materials; however, the sizes of the resonators and the distances among them are much smaller than the targeted wavelength of the radiation. A proper choice of materials and of their arrangement can induce an unusual electromagnetic behavior. In this way, it is possible to conceive e.g. a medium with a negative refractive index (i.e. with simultaneously negative dielectric permittivity and magnetic permeability) allowing one to overcome the diffraction limit in the optical imaging.
Although crystals of TiO2 (rutile) do not exhibit magnetic properties, it is possible to use rutile, due to its high dielectric constant, to create a magnetic response. Layers consisting of randomly distributed TiO2 microspheres exhibit a series of Mie resonances in the terahertz spectral range, where the lowest-frequency one is associated with a strong dispersion in the effective magnetic permeability. W e also developed a new method of measuring unambiguously such a magnetic response [1]. Recently, rigid metamaterials were prepared by embedding TiO2 microspheres into polyethylene. The presented approach shows a way for low-cost massive fabrication of mechanically stable terahertz metamaterials based on dielectric microresonators [2].
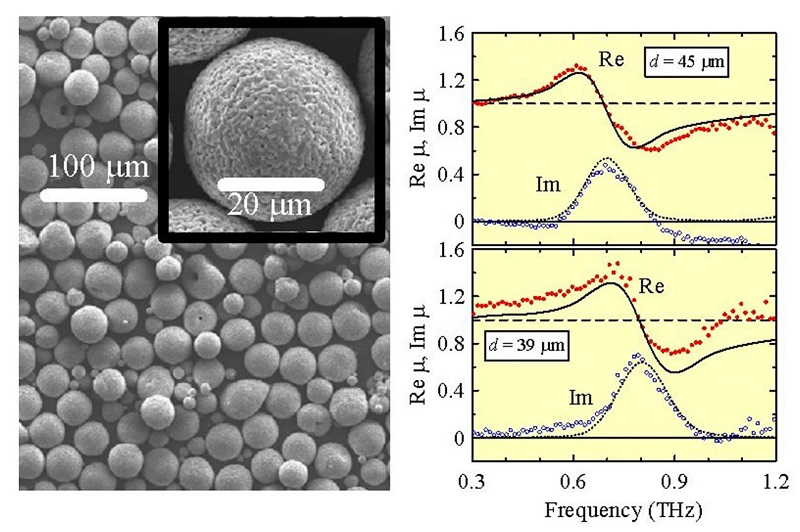
Figure: Left: Scanning electron microscope images of the fabricated TiO2 microspheres before the sorting procedure. Sorting procedure allows one to obtain a narrower distribution of microsphere diameters d. Right: effective magnetic response (real and imaginary part of the effective permeability) of samples with a 10% volume filling fraction of TiO2 microspheres and their sizes d = 45 ± 4 μm and 39 ± 3 μm. Symbols: experiment, lines: results of electromagnetic simulations.
References
[1] H. Němec et al., Appl. Phys. Lett. 100, 061117 (2012).
[2] M. Šindler et al., Opt. Express 24, 18340 (2016).